What is potash?
Potassium (K) is an essential element for all plant, animal and human life. As part of various compounds, potassium makes up about 2.6% of the Earth’s crust by mass and is the 7th most abundant element, similar in abundance to sodium (Na). The chemical symbol for the element, K, derives from kalium, the Latin version of the Arabic word for alkali. In agriculture, potassium is often referred to as potash. This name derives from the ancient practice of obtaining potassium salts by burning wood, extracting the ash with water and evaporating the resulting solution in iron pots – hence “pot-ashes”. The resulting solid would be a mixture of potassium, calcium and magnesium carbonates, chlorides and sulphates. In fact, potash is America’s first industrial chemical for which a patent was issued in 1790 and signed by George Washington. Potash remains an essential product today.
Potash is the common name of various K-rich mined and manufactured salts containing potassium in water-soluble form. Most of the world reserves of K were deposited as seawater in ancient inland oceans. After the water evaporated, the potassium salts crystallized into beds of potash ore, from which potash is being mined today. Over time, as the surface of the earth changed, these deposits were covered by thousands of feet of geological layers.
Evaporite deposits are, therefore, the most important sources of potassium salts in addition to salt lakes and brines because the salts present in these deposits are soluble in water and can be mined and efficiently processed. There are as many as 50 minerals that contain K in brines, salt lakes or salt deposits but only those significantly soluble in water are economically important.
Potash deposits are being mined in Canada, Russia, Belarus, China, Israel, Germany, Chile, USA, Jordan, Spain, UK, Uzbekistan and Brazil. In particular, Saskatchewan in Canada is home to one of the largest potash deposits in the world. Canada has the world’s largest high-grade reserves of potash (accounting for 28 % of the world’s total in 2019) and is the world’s largest producer (31.6 %) and exporter (39 %) of potash.
Most potash mines today are deep shaft mines as much as 4,400 feet (1,400 m) underground. Others are strip mines, having been laid down in horizontal layers as sedimentary rocks composed of evaporite minerals, such as, for example, sylvite (KCl). Potash ores are typically obtained by conventional shaft mining with the extracted ore ground into a powder. Other methods include dissolution mining and evaporation methods from brines. In the above-ground processing plants, KCl is separated from crude (rock) salt (mostly NaCl or MgCl2 or MgSO4.H2O (kieserite)) by thermal dissolution, flotation, and crystallization processes. This can also be achieved by a dry process known as “electrostatic differentiation.” Other potassium salts can be separated by various procedures, resulting in potassium sulfate and potassium-magnesium sulfate. These salts are used to produce a high-analysis potassium fertilizer.
The importance of potash in agriculture and industry
Approximately (90-95%) of all potash production goes into the agriculture sector, where it is used as a plant nutrient. Potassium cannot be substituted by any other nutrient and, therefore, potash has no commercial substitute as a potassium fertilizer source.
The key problem in the optimization of potash processing is the prediction of phase equilibria. This is challenging because of the multiple competing solid phases that may form in Na – K – Mg – Ca – SO4 – H2O systems. The formation of desirable salts is a sensitive function of composition and operating conditions and, therefore, accurate simulation is very useful to pinpoint the optimum conditions.
The following potash deposits and minerals are commercially used for the manufacture of potassic fertilizers:
- Sylvinite, a sedimentary rock made of a mechanical mixture of the minerals sylvite (KCl) and halite (NaCl)
- Carnallite, KCl.MgCl2.6H2O
- Kainite, KCl.MgSO4.3H2O
- Langbeinite, K2SO4.2MgSO4
- Schoenite or picromerite, K2SO4.MgSO4.6H2O
- Syngenite, K2SO4.CaSO4.H2O
- Polyhalite, K2SO4, MgSO4.2CaSO4.2H2O
Fertilizers are plant food. They are combinations of the nutrients that plants must absorb in order to grow. The main elements in fertilizers are nitrogen, phosphorous and potassium (often referred to as N-P-K). There are a variety of “trace” minerals such as copper, manganese, zinc and boron that are also necessary for normal plant growth.
Potassium is an important element because when there is a potassium deficiency in the soil, fertilizers containing potassium are used to help boost crop yields and improve the quality of the plant. Potassium protects plants from extreme temperatures and helps them to fight stress and disease and deter pests such as weeds and insects. K stops wilting, strengthens roots and stems, and assists in transferring food. It activates plant enzymes to ensure plants use water efficiently. Higher levels of potassium in the soil help crops withstand stressful conditions.
Potash is also a component of feed supplements used to grow livestock and enhance milk production. K is an important element of the human diet as it is involved in both cellular metabolism and body functions. It is essential for growth and maintenance of tissues, muscles, and organs, as well as the electrical activity of the heart.
5-10% of potash is used for industrial purposes. The largest industrial use for potash is the manufacture of potassium hydroxide (KOH). KOH is used for industrial water treatment, soap manufacturing and is the precursor of potassium carbonate, several forms of potassium phosphate and other potassic chemicals in a wide variety of industrial processes such as glass making, pharmaceuticals, food processing and the manufacture of textiles. KCl is used in aluminum recycling, in metal electroplating, oil-well drilling fluids, snow and ice melting, steel heat-treating, in medicine as a treatment for hypokalemia, and water softening.
Potash chemistry in the Mixed-Solvent Electrolyte (MSE) model: Modeling nature’s complexity
The OLI simulation framework for electrolytes has long been considered the best candidate for modeling the potash chemistry, but key solid phases and species-species interactions were missing. Therefore, OLI invested heavily in the study of potash systems. Thousands of peer-reviewed experimental data points were collected and critically evaluated to identify the most trustworthy experimental information. Then, the parameters of the Mixed-Solvent Electrolyte (MSE) model were determined via complex multi-stage regressions to obtain the best representation of phase and chemical equilibria. With the new parameters, industrial potash-related processes can be simulated (Wang et al. (2002), Gruszkiewicz et al. (2007)).
The potash chemistry includes salts that are formed by combining four key cations, K+, Na+, Mg+2 and Ca+2 and the two key anions, Cl- and SO4-2. This chemistry is very complex because of a large number of possible combinations of salts and their hydrates in multicomponent mixtures that may form at each temperature. In potash, we are dealing with 8 binary systems (e.g., MgSO4-H2O), 28 ternary systems (e.g., K2SO4-MgSO4-H2O, NaCl-MgSO4-H2O) and numerous multicomponent systems (e.g., K2SO4-MgSO4-CaSO4-H2O). The solids that may precipitate are either single salts (e.g., arcanite: K2SO4) or hydrated single salts (e.g., epsomite: MgSO4.7H2O, bischofite: MgCl2.H2O). In multicomponent systems, double salts (e.g., langbeinite: K2SO4.2MgSO4), hydrated double salts (e.g., schoenite: K2SO4.MgSO4.6H2O) or even hydrated triple salts such as polyhalite (2CaSO4.K2SO4.MgSO4.2H2O) may form. In addition to the formation of multiple solid phases, the complexity of potash chemistry is due to the presence of multiple ionic and neutral species in aqueous solutions due to ion pairing and other chemical equilibria. The most important information in the separation of various solid phases is the difference in their solubilities as a function of temperature and concentration.
Thus, the key challenge is to find the optimum thermodynamic representation of thousands of available (and sometimes not fully consistent) experimental data and to create a set of thermodynamic parameters that reproduces the behavior of all relevant solids with the highest possible accuracy (cf., see Figures 1-5).
The MSE model contains a database of potash species. This database includes thermodynamic properties of all solid phases necessary in fertilizer manufacturing, which were developed based on existing reliable experimental data at various conditions. Examples of phase diagrams for these complex systems are shown in the following figures:

Figure 1 shows experimental solubilities (in molalities, open symbols) in the MgSO4-H2O system as a function of temperature. Magnesium sulfate forms four stable and two metastable hydrates (marked by different colors) in the temperature range from about -4 to 250oC. Solid lines show calculated solubilities of various MgSO4 hydrates and ice (red) in this system.
To ensure healthy and nutritious plant growth, an adequate supply of potassium must be maintained in the soil. Mineral fertilizers can be designed to meet the nutritional needs of specific plants and soils. Today, potassium is available in a range of fertilizers. Some contain only K, while others contain two or more nutrients. Muriate of potash (MOP, potassium chloride, KCl) accounts for about 95% of all potassium fertilizers used in agriculture.
In some of the world’s potash reserves, sylvinite (a mixture of KCl and NaCl) is being depleted and there is a risk of shortages (Tavares et al. (2017)). Therefore, the process of separation of KCl from different potash deposits has to be explored. In separation of salts, the knowledge of solid-liquid equilibria in multicomponent systems is extremely important. Figure 2 presents experimental (symbols) and calculated (solid and dash lines) solubilities in the ternary KCl-K2SO4-H2O system at different temperatures marked with various colors. Depending on temperature and the coexisting salt concentrations, four solids may precipitate in this system: ice, KCl, K2SO4.H2O and K2SO4.

Sulphate of potash (SOP, potassium sulphate, K2SO4) and langbeinite (K2SO4.2MgSO4) or K-Mag (potassium-magnesium sulphate) are often included as part of mixtures, blends and compound fertilizers.
In areas with low rainfall, the chloride in the standard fertilizer product can accumulate and give rise to some salinization of the soil. In these situations, SOP is the preferred fertilizer because it is chloride-free, but it contains two plant nutrients, potassium and sulfur. To date, the main natural sulfate salts that have been successfully processed to manufacture SOP are kainite (KCl.MgSO4.3H2O), kieserite (MgSO4.H2O) and langbeinite (K2SO4.2MgSO4).
On the other hand, langbeinite and also SOP are favored by producers of chloride-sensitive crops (e.g., potatoes, soybean, tobacco, fruit and vegetables) with high market value that require other essential nutrients. Langbeinite is a unique source of plant nutrition, since three essential nutrients combine naturally into one mineral. It provides a readily available supply of potassium, magnesium and sulfur to growing plants. Soluble Mg has been documented to improve photosynthesis, enzyme activation, and grain yield. K-Mag is a unique nutrient source that features low chloride, water soluble nutrients, and does not affect soil pH.
Therefore, the knowledge of solid-liquid equilibria in the ternary K2SO4-MgSO4-H2O system is essential in the process of obtaining these two fertilizers (SOP and langbeinite) from various potash deposits.
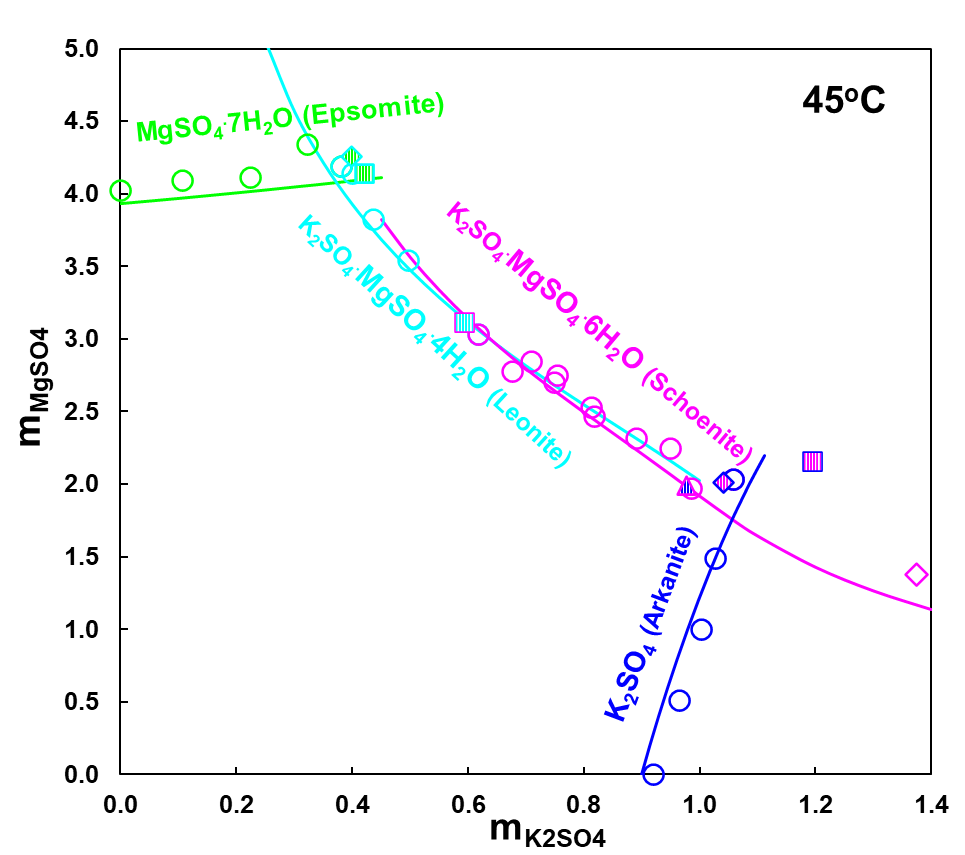
Figure 3 shows solubilities of various solid compounds formed by K2SO4 and MgSO4 in water at 45oC. Depending on K and Mg sulfate concentrations, four different solids form in this system: K2SO4 (arkanite), K2SO4.MgSO4.6H2O (schoenite), K2SO4.MgSO4.4H2O (leonite) and MgSO4.7H2O (epsomite). However, Figure 4 illustrates that at 75oC, schoenite is not stable anymore, a new phase, langbeinite forms and the heptahydrate of MgSO4 is replaced by its monohydrate, kieserite. Calculated solubilities represented by solid lines in Figures 3 and 4 show that the MSE model reproduces experimental data in this system with a high accuracy.
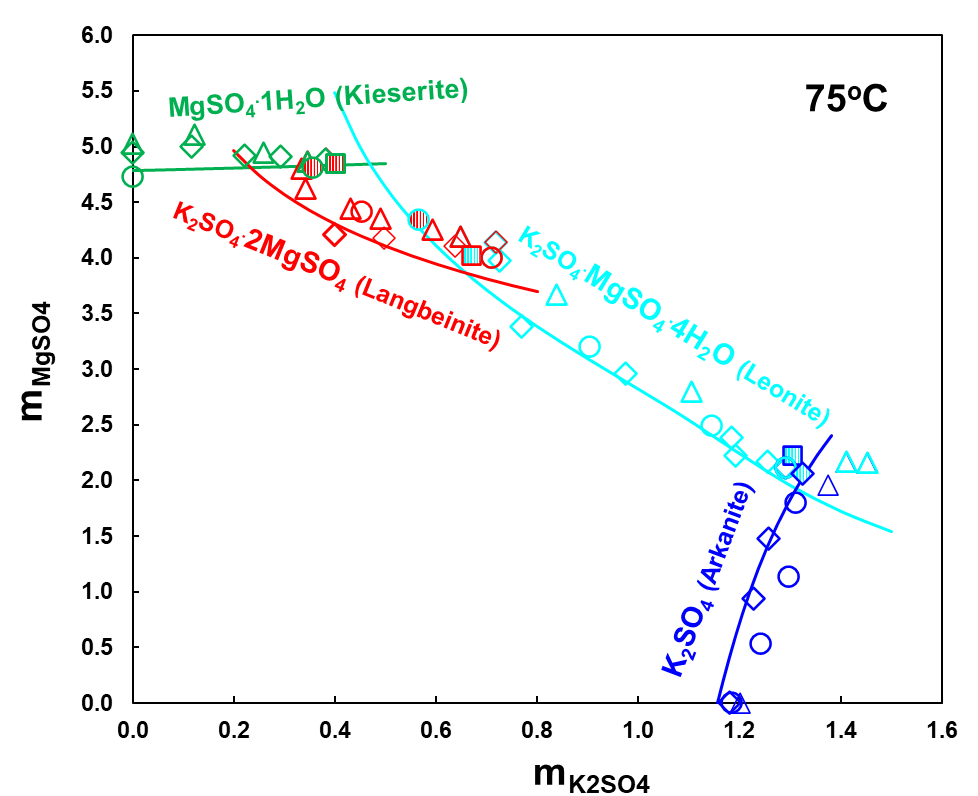
For quaternary reciprocal systems, the results are best represented using Jänecke diagrams. In Jänecke diagrams, the “dry salt” compositions (water excluded) are used for each of the ion components to represent the stability areas of the solids. Each line in such diagram thus represents the border between two stable (or metastable) solids and the invariant points where three lines intersect represent the conditions under which 3 solids coexist. Such diagrams are very useful for interpretation of results in evaporation of water from brines, mineral extraction from salt lakes and sea waters, and crystallization. They are a great tool to determine key operating parameters to define the optimum process conditions to minimize costs and optimize production.
Figure 5 demonstrates a very complex Jänecke diagram for the Mg-K-SO4-Cl-H2O system at 35oC. The “dry-salt” compositions are denoted by x-K, x-Mg, (1-x)-K2 and (1-x)-Cl2. Ten various solids (single and hydrated salts and double salts hydrates) form in this system: KCl, K2SO4, MgSO4.7H2O, MgSO4.6H2O, MgSO4.1H2O, MgCl2.6H2O, K2SO4.MgSO4.6H2O (schoenite), K2SO4.MgSO4.4H2O (leonite), KCl.MgSO4.3H2O (kainite), KCl.MgCl2.6H2O (carnallite). Different colored lines represent the borders between two neighboring stable salts and these pairs of salts are marked with the same colors as corresponding lines in the legend. For example, the light green line is the border between stable schoenite and MgSO4.7H2O and the gray line is the border between leonite and kainite. The intersection between dark blue, brown and pink lines represents concentrations under which K2SO4, KCl and schoenite coexist at 35oC.
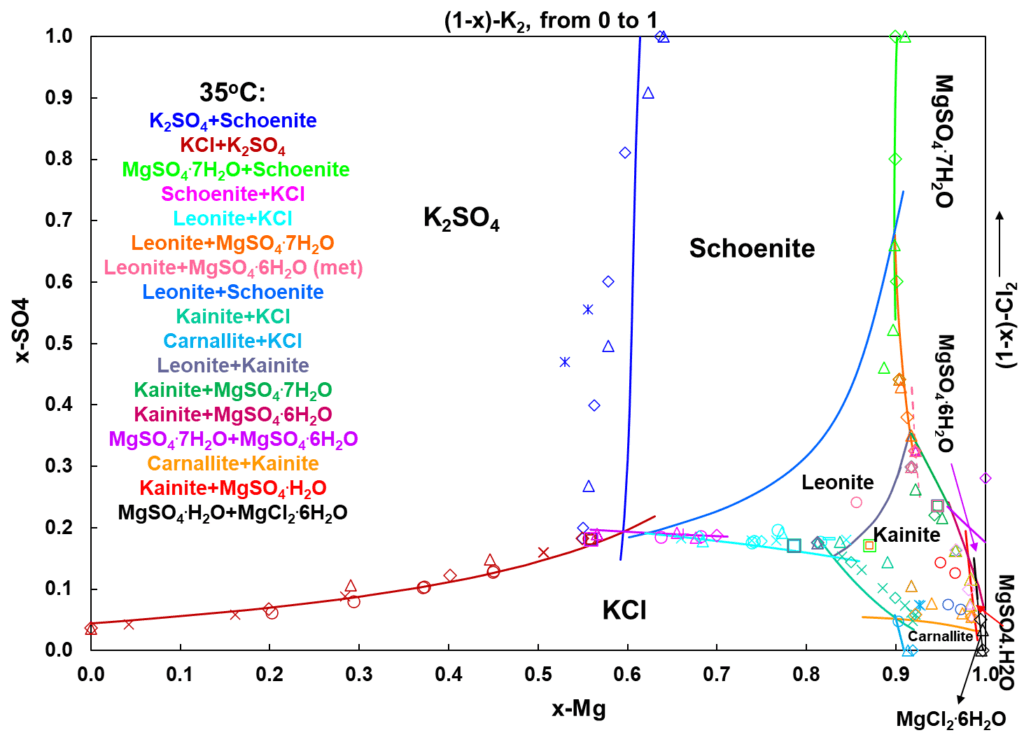
What tools are available for simulating potash chemistry?
OLI System’s thermodynamic property package, which implements the MSE model, is available in OLI Studio V11 and OLI Flowsheet ESP V11.
Contact OLI for more information or to schedule a meeting with an OLI expert.
References
- Wang, P.; Anderko, A. and Young, R. D. “A speciation-based model for mixed-solvent electrolyte systems,” Fluid Phase Equilbria 2002, 203, 141-176
- Gruszkiewicz, M.; Palmer, D. A.; Springer, R. D.; Wang, P. and Anderko, A. “Phase behavior of aqueous Na-K-Mg-Ca-Cl-NO3 mixtures: Isopiestic measurements and thermodynamic modeling,” Journal of Solution Chemistry 2007, 36, 723-765
- Tavares, J.A.; Moura, L.F.; Bernardo, A.; Giulietti, M. “Crystallization and separation of KCl from carnallite ore: Process development, simulation, and economic feasibility,” Chemical Industry and Chemical Engineering Quarterly 2017, 24(3), 239-249