Selenium in the environment
Selenium (Se) is a metalloid found in Group VIA of the periodic table and located between elemental sulfur (S) and tellurium (Te). It has similar chemical properties to sulfur due to its analogous electron distribution, which can make remediation in the presence of S challenging. It was first identified in 1817 by Jöns Jakob Berzelius and Johan Gottlieb Gahn while studying sulfuric acid in lead-lined chambers. The name of the element is derived from the Greek word for the Moon goddess, Selene, since tellurium, which was discovered earlier had been named in for the Earth god, Tellus in Latin.
Se is a naturally occurring element in the Earth’s crust at a concentration of 50 ppb, making it the 67th most abundant element. It is redistributed in the environment through multiple natural and anthropogenic processes. The major geological sources of Se include crustal rock such as black shale, phosphate rocks, coal, coal ash and soils. It can also be found in rare Se-containing minerals occurring together with various metal (such as Cu, Pb, Ni, Fe, Co, Zn) sulfides. Natural processes that redistribute Se include volcanic activity, terrestrial weathering of rocks and soils, wildfires, and volatilization from plants and water bodies. Se is redistributed from the various enriched geological sources into aquatic, sedimentary, atmospheric, and terrestrial environments. As a result, Se is present in the environment in both inorganic and organic forms, and in the solid, liquid, and gas phases. Regionally, human activity is a major factor in the mobilization of selenium. Mining, fossil fuel combustion, oil refining, and discharge of seleniferous drainage water from irrigated agriculture are all anthropogenic sources of Se in aquatic systems.
Industrial applications of selenium
Se finds many applications in diverse industries. Specifically, it is used in the manufacture of glass and pigments (50% of consumption), metal alloys, petroleum products, new chemicals, textiles, photographic emulsions, cosmetics, and medical therapeutic formulas. Se substitutes lead in ceramics, plumbing fixtures, solder, brass (with bismuth) and other metal alloys.
Due to its good photovoltaic and photoconductive properties, Se is widely used in photocells, light meters, solar cells, toners, photocopiers, and other electronic devices. In the refining of manganese (Mn), the addition of selenium dioxide (SeO2) as a substitute for sulfur dioxide (SO2) reduces power consumption and increases Mn yield. One of the most promising systems for energy storage in the family of lithium batteries is the lithium–selenium (Li–Se) battery, which is an alternative to the Li-S battery with an advantage of high electrical conductivity.
Selenium dilemma: Beneficial and damaging effect on living organisms
The effect of Se on living organisms can be both beneficial and damaging. At low levels, Se is an essential element for the normal cellular function of living organisms. In humans, it is a component of several major metabolic pathways including thyroid hormone metabolism, antioxidant defense system and immune function. Dietary selenium comes from meat, fish, eggs, nuts, grains, vegetables, and fruits. Deficiency occurs when the daily intake falls below 40 μg/day. This happens when the food is grown in soils with low Se level. There is substantial evidence that the selenium deficiency affects the health of the heart and nervous system, interferes with reproduction and gestation, and negatively influences immunocompetence.
Although Se is an essential element for all organisms, introduction of Se into the environment from natural and anthropogenic sources can lead to its increased concentrations in surface water, groundwater, soils, and vegetation. Consequently, Se can bioaccumulate and may become toxic to sensitive aquatic life, birds, and mammals including humans resulting in many short- and long-term health effects such as damage to the nervous and circulatory systems, liver and kidney damage and decline in reproduction. When the tolerable upper daily intake limit is exceeded, selenosis can occur; the typical symptoms include gastrointestinal disorders, hair loss, sloughing of nails, fatigue, irritability, cardiac arrhythmia, and neurological damage. The margin between essentiality and toxicity of Se in adult humans is the narrowest of all trace elements, making the risk of negative impacts from environmental contamination extremely high. Toxic levels are not well established and are reported to vary from 2 to 7 times the nutritionally required Se intake (which is equal to 55 μg Se/day).
Selenium as a water contaminant
There is, probably, no chemical contaminant for which there are more contradictions about ecological risks in aquatic environments than selenium. Selenium pollution is a worldwide phenomenon and is associated with a broad spectrum of human activities, ranging from the most basic agricultural practices to the most high-tech industrial processes.
Selenium can be released into the environment from mining activities, power generation, especially mining and combustion, oil transport, refining and utilization, and agricultural irrigation of Se-rich soils. In mining wastewaters, Se is commonly found in concentrations ranging from 3 to more than 12000 μg/L. Mining of coal, precious metals (gold, silver) and metallic sulfides is the key contributor to exposing Se-bearing compounds to air and water. This mobilizes selenium into aquatic systems where it bioaccumulates in the food chain escalating its hazardous effects.
A well-known example of anthropogenically-caused Se toxicity occurred between 1974 and 1986 in Belews Lake in North Carolina as a result of a discharge of wastewater containing 150-200 μg Se/L from a coal-fired power plant. At that time, selenium found predominantly as selenite (Se(+4)) in fly ash, was associated with effluents being discharged from a coal-fired power plant which caused extirpation of 19 resident fish species.
Another well documented example of Se toxicity is the Kesterson Reservoir in California, where subsurface drainage of agricultural irrigation water that reached concentrations as high as 4200 μg/L (predominantly as selenite), resulted in devastating impacts on fish and wildlife populations dependent on those habitats. The most notable impacts at Kesterson were the reproductive effects to birds using the wetlands (Beatty and Russo, 2014). High rates of reproductive failure and deformed hatchlings were reported, as well as signs of acute poisoning in adult birds in the most contaminated areas. Selenium poisoning in birds can be passed from parents to offspring through the egg and it may persist for many generations.
Selenium guidelines for drinking, fresh, marine, and salt water
Selenium contamination continues to attract the attention of natural resource and water quality regulators around the world. EPA has set the Se standard for drinking water at 50 ppb (or μg/L) Se to protect consumers served by public water systems from the effects of long-term, chronic exposure to selenium.
For comparison, the World Health Organization (WHO) and the European Commission (EC) have set the provisional guideline for Se in drinking water at 40 ppb and 10 ppb, respectively. EPA included a 5-ppb discharge limit for Se for freshwater, 300 ppb for marine water and 71 ppb for saltwater as a part of the Clean Water Act, which is a water quality criterion for protecting aquatic life, human health, and ecosystem. In contrast, Canada’s marine and freshwater guidelines are 2 ppb. The EPA’s freshwater criterion has a lower guideline of 2 ppb for some wetland environments in California. EPA is also responsible for developing ELG (effluent limitations guidelines) for controlling industrial wastewater discharges.
Various physical, chemical, and biological methods were developed to meet the above criteria of the required level of selenium in water. It is worth noting that the determination of low levels of different inorganic selenium species in water is an analytical challenge. The speciation and solubility of Se in aqueous environments plays a critical role in the effectiveness of any approach for removal, especially to low levels. Very often, these methods require a deep knowledge of aqueous chemistry.
Selenium chemistry in the Mixed-Solvent Electrolyte (MSE) model
In aqueous environments, Se exists in four oxidation states:
- Se(-2): H2Se and selenides, MeSe, where Me = Cu, Pb, Co, Fe, etc.
- Se(0): Elemental selenium
- Se(+4): H2SeO3, SeO2 and selenites, MeSeO3, where Me = Na, Mg, Fe, Mn, Zn, etc.
- Se(+6): H2SeO4, SeO3 and selenates, MeSeO4, where Me = Na, Mg, Zn, Mn, Pb, etc.
Table 1 shows oxidation states, solubilities, sorption ability, reduction kinetics and toxicity of Se species.
Selenates are very soluble and can exist only in oxidizing media. Selenates are the most common form of Se in wastewater. They have weak sorption capabilities meaning that the adsorbent materials such as flocculating polymers, carbon, ion exchange, media filters, and other similar technologies have little effect. Selenates also have very slow reduction kinetics. Special bacteria can reduce selenates to selenites and elemental selenium, but the process can be slow and requires large amounts of nutrients. Selenites are present at moderately reducing conditions and are also very soluble and can be adsorbed more easily. Their reduction kinetics is faster than that of selenates. Selenites are not as common in wastewater as selenates but are more toxic. Elemental Se is a naturally occurring element. It has very low solubility and slow oxidation-reduction kinetics forming a colloidal suspension in water. Therefore, it can be filtered and adsorbed. It can be also oxidized by microorganisms to sediment-bound selenites and trace amounts of selenates. Selenides are soluble but can be precipitated with various metals. They have weak sorption capabilities and are highly toxic similar to selenites.
Speciation of Se in aqueous solutions depends on temperature, pH, redox potential, solubilities of selenium salts, complexing ability of aqueous ligands, and biological activity and reaction kinetics. Since different chemical species of selenium have differing biological reactivity and availability and chemical and geochemical properties, the knowledge of selenium speciation is important to understand its fate and environmental effects.
The Mixed-Solvent Electrolyte (MSE) model contains a database of Se species. Thermodynamic properties of selenides, elemental Se, selenites and selenates were developed based on existing experimental data. Examples of predictions for important Se-containing mixtures are shown in the following figures.
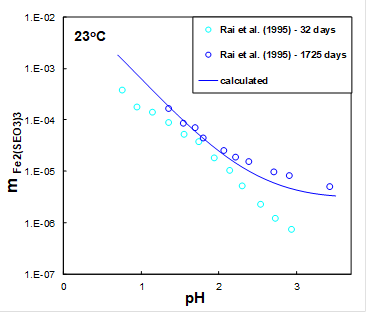
In oxidizing sedimentary environments, Se tends to associate mainly with iron (Fe) oxides in adsorbed or precipitated forms. The existence of ferric selenite hexahydrate (Fe2(SeO3)3.6H2O) has been known for many years. Figure 1 shows the solubility of iron selenite hexahydrate in water after 32 and 1725 days (blue symbols) measured as a function of pH adjusted by HCl at room temperature. It can be seen from Figure 1 that after 32 days, the system did not achieve equilibrium. The MSE model (solid line) shows that the solubility of iron selenite hexahydrate decreases with pH in agreement with experimental data.
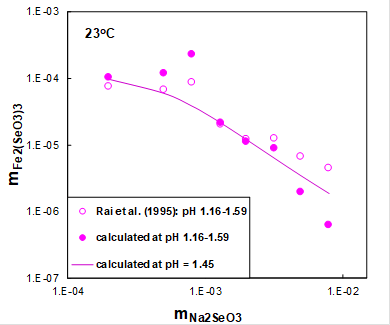
Figure 2 shows the solubility of ferric selenite hexahydrate (open symbols) as a function of sodium selenite concentration at room temperature and constant initial pH. The initial pH in this system was equal to 1.5 and was adjusted by HCl. Solid symbols show the solubility in this system at equilibrium pH values ranging from 1.16 to 1.59 calculated using the MSE model. Solid line shows calculated solubilities at an average pH.
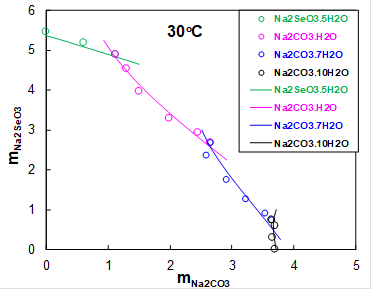
Sodium selenite is mainly used in the manufacture of colorless glass. It was of interest to explore what solid phases precipitate in the ternary Na2SeO3-Na2CO3-H2O system. Carbon dioxide is always present in the atmosphere, thus acting as a source of inorganic carbon. Figure 3 shows the solubility of Na2SeO3 as a function of Na2CO3 concentraton at 30oC. At low Na2CO3 concentrations, sodium selenate pentahydrate precipitates (green symbols). At molalities of Na2CO3 above 1, sodium carbonate monohydrate (pink symbols), heptahydrate (blue symbols) and decahydrate (black symbols) precipitate. The MSE model accurately predict solubilities of all solids in this system.
Raman spectral studies of aqueous solution of selenic acid (H2SeO4) were conducted at 25oC providing the distribution of aqueous species in this system. Figure 4 shows that at very low concentration (in mole fraction) of selenic acid, selenate ion (SeO4-2, red symbols) dominates and its concentration decreases with an increase of selenic acid and achieves a minimum at the mole fraction of selenic acid around 0.3. At the same time, the concentration of hydrogen selenate ion (HSeO4–, blue symbols) increases and achieves its maximum for the mole fraction of selenic acid equal to ~3. At this concentration, the amount of the neutral selenic acid species (H2SeO4, green symbols) has its minimum and gradually increases with the selenic acid concentration achieving its maximum at the mole fraction of selenic acid at about 0.92. The yellow line shows increasing concentrations of the aqueous neutral selenium trioxide (SeO3) at the mole fractions of selenic acid above 0.8. The MSE model predicts the speciation in this system with great accuracy as shown with the red, blue, green, and yellow solid lines in Figure 4.
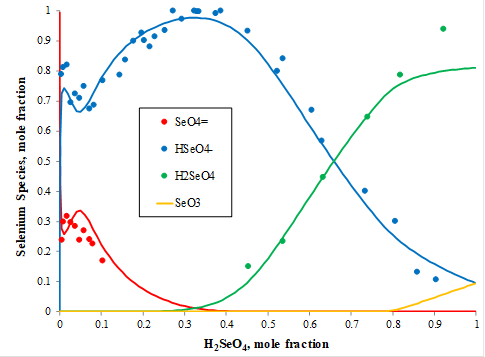
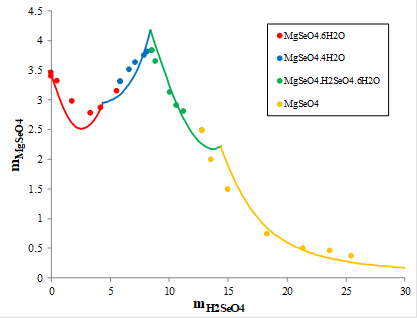
Magnesium is widely available in nature. It was of interest to explore the solid-liquid equilibria in the MgSeO4-H2SeO4-H2O system. Figure 5 shows the solubility of magnesium selenate (MgSeO4) in aqueous selenic acid measured at 30oC. At concentrations of selenic acid below 5.6 molal, magnesium selenate hexahydrate (MgSeO4.6H2O, red symbols) precipitates and then it is replaced by magnesium selenate tetrahydrate (MgSeO4.4H2O, blue symbols) at higher selenic acid concentration. At the molality of selenic acid equal to about 8 m, a double acid salt hexahydrate (green symbols, MgSeO4.H2SeO4.6H2O) becomes more stable and precipitates. Then, it is replaced by anhydrous magnesium selenate (MgSeO4, yellow symbols) at selenic acid concentration above 13 m. The red, blue, green, and yellow solid lines show the calculated solubilities in this complex system, which were obtained using the MSE model.
What tools are available for simulating Se chemistry?
OLI System’s thermodynamic property package, which implements the MSE model, is available in OLI Studio V10 and OLI Flowsheet ESP V10.
The OLI software can be used to gain understanding of the solution chemistry of selenium that is associated with various selenium removal processes. Such processes include reduction of selenites and selenates, iron co-precipitation, ion exchange, removal on activated alumina and various biological treatment processes (cf. the review by Sandy and DiSante, 2010). For those applications, it is necessary to know the oxidation state, speciation, and solubility of Se species, which can be obtained from OLI’s MSE model.
Contact OLI for more information or to schedule a meeting with an OLI expert.
References
- J.M. Beatty and G.A. Russo, “Ambient water quality guidelines for Selenium Technical Report Update,” Water Protection and Sustainability Branch Environmental Sustainability and Strategic Policy Division, British Columbia Ministry of Environment, April 2014
- Sandy, C. DiSante, “Review of Available Technologies for the Removal of Selenium from Water” Final Report for North American Metals Council, CH2M Hill, 2010, https://www.namc.org/docs/00062756.pdf